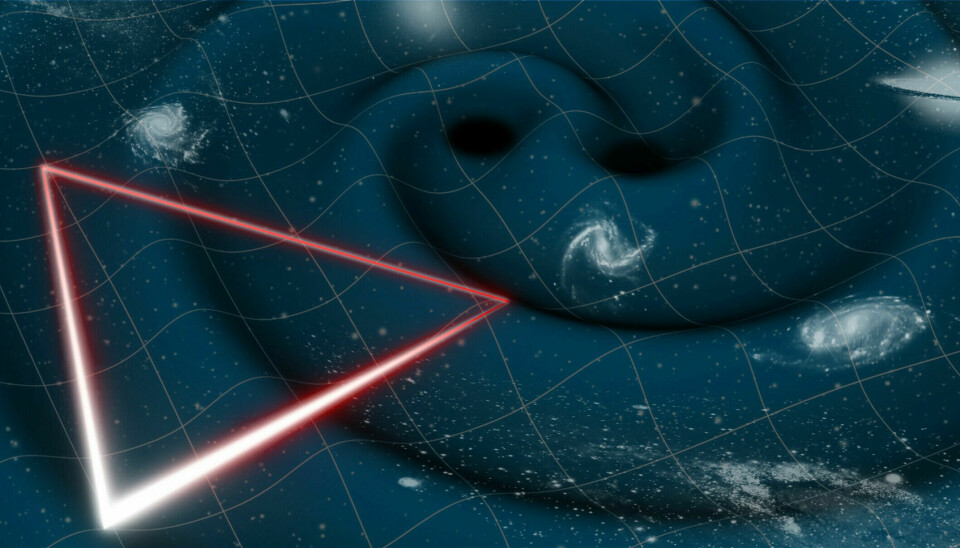
Giant lasers will capture waves in space: The idea was considered crazy 30 years ago
“Sometimes dreams do come true,” says Helena Kolesova, a particle physicist at the University of Stavanger.
The European Space Agency (ESA) has decided that the Laser Interferometer Space Antenna (LISA) instrument will be built. The work starts in January 2025, ESA reports.
LISA consists of three instruments that will be sent into space. They will point lasers at each other in a perfect triangle formation.
Each side of the triangle will be 2.5 million kilometres, more than six times the distance from Earth to the Moon.
Through this method, researchers aim to detect gravitational waves, which are ripples in spacetime.
As these waves gently rock the space illuminated by LISA’s lasers, space expands and contracts ever so slightly.
With the lasers, this difference in distance can be detected.
We are talking about a magnitude of a few picometres, a unit of measurement at the atomic level. A helium atom has a diameter of 62 picometres.
Sci-fi instrument
Most viewed
“The idea of LISA was crazy and very sci-fi 30 years ago,” Helena Kolesova says.
She is thrilled that ESA has given the go-ahead for the project.
“My first thought was that I was very happy for everyone who has worked on preparing the LISA mission for 20 to 30 years,” she says.
An instrument called LISA Pathfinder, which proved that the technology could work as intended, has previously been sent up.
Kolesova is herself a part of the LISA collaboration, but she works with theory. She explores how gravitational waves from the early universe can reveal new physics, such as the nature of dark matter.
There are also others at the University of Stavanger who study how data from LISA can be utilised. Such as Germano Nardini, one of the leaders of LISA's cosmology working group. He is "the heart of LISA in Norway," says Kolesova.
First detected in 2015
The construction and preparations are expected to take ten years, and the plan is for LISA to be launched in 2035.
LISA will follow behind the Earth in orbit around the Sun. In each of the three instruments, there will be two cubes made of gold and platinum. The boxes are slightly smaller than a Rubik's cube.
The distance between the boxes is measured using the lasers.
Gravitational waves are formed when large masses in the universe rotate closely around each other, such as black holes.
Since 2015, ground-based detectors have registered gravitational waves from a number of mergers between black holes and from other events.
The LIGO observatories in the USA have two four-kilometre-long arms in an L-shape. Lasers are used to measure tiny deformations of space caused by gravitational waves.
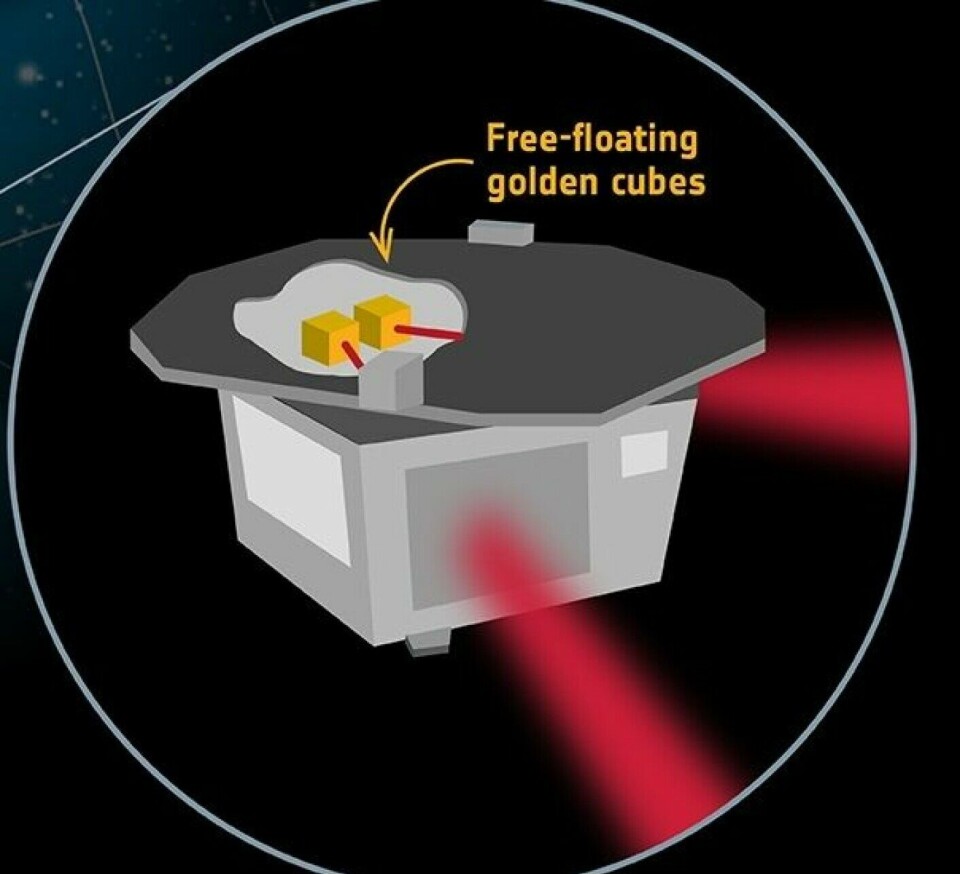
The distance will vary somewhat
LISA works a bit like LIGO, according to Kolesova.
Laser beams are sent between satellites and combined. If one of the arms has changed length, there will be interference. The waves in the light do not match perfectly.
But how is it possible to keep LISA sufficiently still to measure distance differences the size of an atom?
Fortunately, a little variation in distance is fine.
“The distances will change a bit, but it will happen in a controlled way,” she says.
Researchers know how these slow changes in distance will play out and can subtract these effects. What remains is the effect of gravitational waves.
The triangular shape is clever, according to Kolesova. This is useful in data analysis, because a lot of noise can be filtered out. It also helps to determine where the gravitational waves came from.
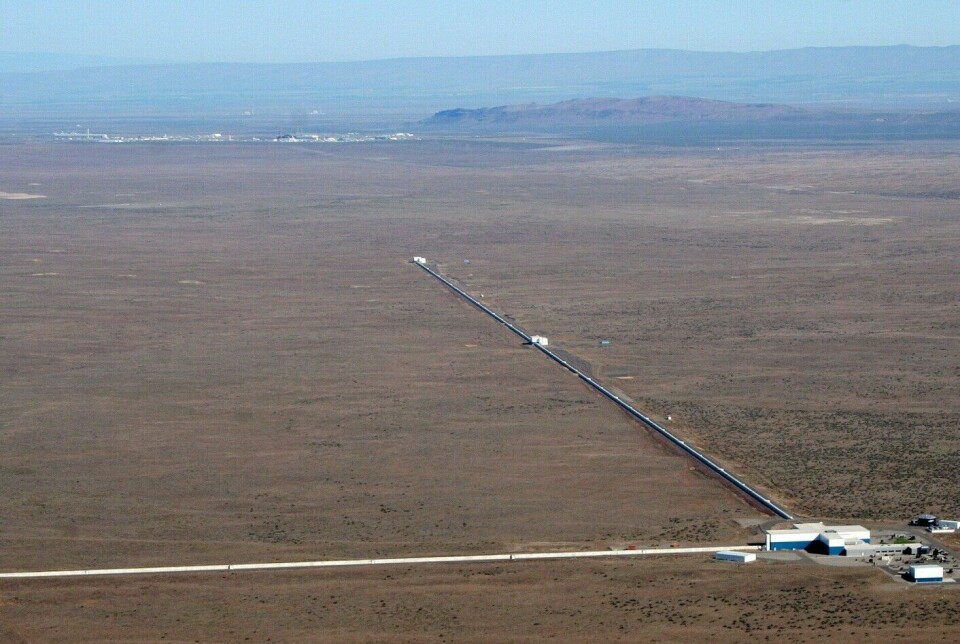
Will detect larger waves
Why send up an observatory for gravitational waves in space when we already have several of them on Earth?
LISA will be able to detect gravitational waves at different frequencies than the ground-based detectors, Kolesova explains.
Electromagnetic radiation has different wavelengths. Visible light has a relatively short wavelength, while radio waves have a long wavelength.
Different telescopes are used to study electromagnetic radiation with different wavelengths.
Similarly, different detectors are needed to capture gravitational waves with short and long wavelengths.
LISA has much longer arms than the ground-based observatories. This allows the instrument to detect gravitational waves with much longer wavelengths.
Aftereffects of galaxies that collided
LIGO captures signals from black holes or neutron stars in the final phase before merging.
LISA, on the other hand, will have the capability to witness this cosmic dance much earlier, when the black holes are rotating further apart, emitting gravitational waves with longer wavelengths.
“LISA will observe the same events as LIGO, but at an earlier stage,” Kolesova says.
This means that LISA can alert to an event that LIGO will detect weeks or months later. It also allows for the pre-positioning of ordinary telescopes to observe the event and detect any radiation emitted during the collision of objects.
LISA will also be able to detect larger and even more violent events than LIGO: Namely, supermassive black holes colliding.
“These massive black holes are thought to be in the centre of galaxies,” Kolesova says.
Mergers between supermassive black holes will produce a lot of gravitational waves and can be ‘heard’ from extremely long distances.
“If you look far out into the universe, you also look far back in time. This is because gravitational waves have a certain speed, the same speed as light,” she says.
This means that mergers between galaxies early in the universe can be registered, thus providing more information about the development of the galaxies.
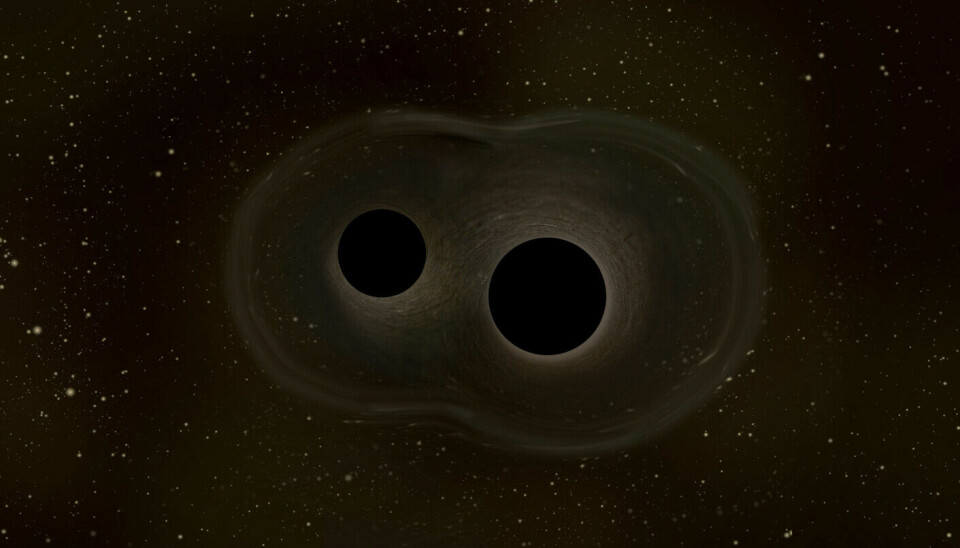
Expansion of the universe
There are also other measurements that can be made with LISA. Some are eager to see what LISA can reveal about the speed of the universe's expansion. This speed is called Hubble's constant.
“But there’s a problem. If you measure how fast the universe is expanding in our local universe, the value is slightly different from if you measure it from the cosmic microwave background,” Kolesova says.
The cosmic microwave background represents the earliest light in the universe, emanating from all directions. According to theory, this light was released 380,000 years after the Big Bang, when electrons bound to atomic nuclei and light could travel freely.
LISA can help determine which measurement of the universe's expansion is correct.
“It may even be that both measurements are correct and that our theory needs to be changed,” she says.
The first years are dark
Then we come to what Kolesova is most excited about.
We cannot see light or electromagnetic radiation that is older than the cosmic microwave background. The first 380,000 years of the universe's history are shrouded in darkness.
For gravitational waves, the same limitation does not apply. If gravitational waves were formed in the early universe, these can still be detected as ripples in spacetime.
“There might be something similar to the cosmic microwave background, known as a stochastic background of gravitational waves,” says Kolesova.
Physics beyond the Standard Model
The Standard Model outlines the fundamental building blocks for everything that exists. It explains how atoms and particles interact and behave. The theory works extremely well.
“However, when we look out into the universe, there are some problems that we can’t explain with the Standard Model. One of these is dark matter,” Kolesova says.
Dark matter appears on various scales in the universe. Galaxies are heavier than they appear. One explanation is the presence of invisible matter within the galaxy.
This dark matter seems to interact minimally with ordinary particles, yet it has mass and is affected by gravity.
Gravitational waves from the early universe could provide insights into dark matter and other particles not accounted for in the Standard Model.
Echoes from the dawn of the universe
For instance, gravitational waves may have been produced during so-called phase transitions in the early universe. A phase transition occurs when a substance changes properties, like water transitioning from liquid to vapour.
Phase transitions occurred in the early universe, says Kolesova.
One such transition happened when the universe cooled from a hot soup, leading to the formation of protons and neutrons. There was also a phase transition when particles acquired mass through the Higgs field.
However, these were not violent phase transitions, Kolesova notes, and they did not generate gravitational waves.
If, on the other hand, unknown particles were involved, violent phase transitions and gravitational waves could have occurred.
Researchers will therefore listen for such a background hum of gravitational waves from the early universe.
“These would be gravitational waves coming from all directions. They will be difficult to detect, because of the constant noise,” she says.
What has been discovered with pulsars?
Last year, news emerged that researchers have seemingly detected such a hum using pulsars.
Pulsars are neutron stars that spin rapidly. They cast pulses of light towards Earth like a ticking clock. These are also used to detect gravitational waves.
“This is a hot topic at the moment,” says Kolesova.
Researchers have found signs of a stochastic gravitational wave background in the data sets from pulsars, she says.
“But we don't know the origin yet. The most conservative interpretation is that it originates from supermassive black holes merging,” she says.
So, it might be that the background hum is the remnants of numerous collisions between supermassive black holes over time.
“Even if the signal comes from black holes, it’s interesting. People did not expect the signal to be so strong,” she says.
———
Translated by Alette Bjordal Gjellesvik
Read the Norwegian version of this article on forskning.no